Question
A pipe is tapered so that the large end has a diameter twice as large as the small end. What must be the gauge pressure (the difference between pressure at the large end and pressure at the small end) in order for water to emerge from the small end with a speed of 12 m/s if the small end is elevated 8 m above the large end of the pipe?
Final Answer
Solution video
OpenStax College Physics for AP® Courses, Chapter 12, Problem 10 (Test Prep for AP® Courses)
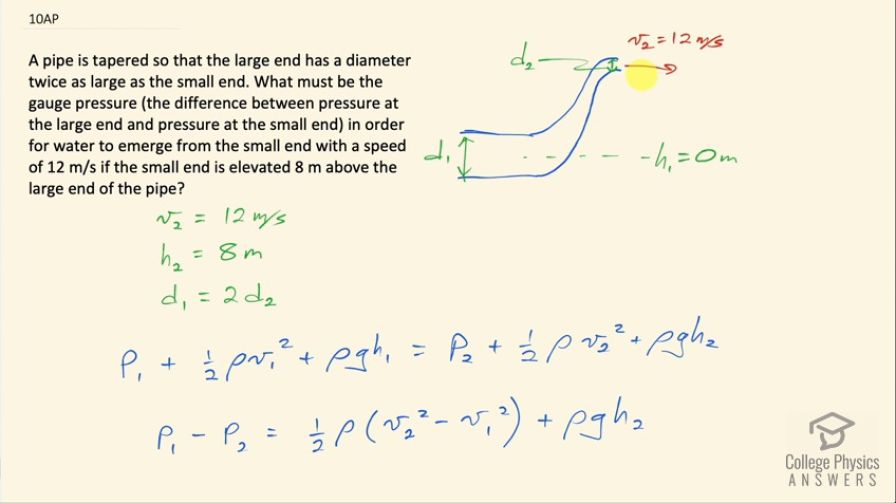
vote with a rating of
votes with an average rating of
.
Calculator Screenshots
Video Transcript
This is College Physics Answers with Shaun Dychko. A tapered pipe is elevated such that the small diameter end is 8 meters above the beginning end so this is h 2—8 meters here— and the water is coming out of the pipe at a speed of 12 meters per second and the diameter here is labeled d 2 and the diameter here is labeled d 1 and we are told that the large diameter is 2 times the small diameter and our job is to figure out what is the difference in pressure between the first and second points here? It's called the gauge pressure, in other words. Bernoulli's principle says that the pressure at the beginning plus one-half times the density of water times its speed there squared plus density of water times g times its height but its height is zero—we'll take this to be the reference level... this term is going to disappear— all of this equals the pressure at the second position plus one-half density times the speed of the fluid there squared plus density times g times height two. We are looking for P 1 minus P 2— this is the gauge pressure— so subtract P 2 from both sides and then get rid of this term from the left and then bring it to the right hand side by subtracting one-half ρv 1 squared from both sides and then factor out the one-half ρ from this term and this term and we are left with one-half ρ times v 2 squared minus v 1 squared plus ρgh 2. Now we can't solve this because we don't know what v 1 is— the speed of the fluid at the beginning of the pipe— so we need to look at the continuity equation this is a conservation of mass equation for this context and it says that the cross-sectional area of the first position times the speed of fluid there equals the cross-sectional area at the second position times the speed of the fluid there. And since it's a circular cross-section we assume because it uses the word 'diameter,' we can say that the area one is π times the radius at position one squared and I am writing radius as diameter divided by 2 and that v 1 is multiplied by that and then on the right-hand side, we have the area at the position two, π times diameter two over 2 squared times v 2 and the π and the denominator 4 cancels on both sides so we multiply both sides by 4 over π the 2 is squared down there that's how that becomes 4 okay... and we are left with d 1 squaredv 1 equals d 2 squaredv 2 and we know that d 1 is twice d 2 so we can make a substitution for that and write d 1 as 2d 2 instead. And we can divide both sides by d 2 squared and then this 2 squared makes 4 so we have 4v 1 equals v 2 in which case v 1 is v 2 divided by 4 so now we can replace this unknown v 1 with v 2 over 4 and that's what we do here and then we plug in numbers. So that's one-half times the density of water, which is 1000 kilograms per cubic meter times 12 meters per second squared minus 12 over 4 squared plus the density times gravitational field strength times the height at position two of 8 meters and this is a gauge pressure or pressure difference in other words of 1.5 times 10 to the 5 newtons per square meter.